Spectrum strategy is becoming an ever more crucial factor
Explosive growth in wireless data services is once again transforming an industry well-accustomed to change. The rapid growth in traffic of the 2000s, driven predominantly by voice subscriber additions, is now being followed by an even more dramatic increase in data traffic, as smart phone market penetration accelerates and a flourishing ecosystem of smartphone apps drives explosive growth in the amount of data consumed per subscriber.
To continue to support this growth, spectrum strategy is becoming an ever more crucial factor in determining a wireless operator’s success. Spectrum strategy discussions usually center on opening new bands for terrestrial wireless services, but it should be emphasized that the core issue is providing sufficient and pervasive network throughput to keep pace with the acceleration in demand. This is a question not only of adequate spectrum, but also of maximizing spectral efficiency, i.e. to support continued growth in demand for data services, operators must increase spectrum holdings, and also maximize the through-put per unit of available bandwidth per unit of coverage area (the area spectral efficiency, typically measured in kbps/Hz/km2).
3- and 4G wireless data air interfaces have dramatically improved the theoretical spectral efficiency of wireless networks, to the point that LTE can exceed 80% of the Shannon limit in highly-idealized cases. However, even the most elaborate modulation schemes are susceptible to degradation due to interference. As any mobile service subscriber knows, the gap between real world performance and the theoretical ideal is often large. In many cases, this is due to the presence of interference.
Interference can originate from a myriad of sources, but the impact to the end user is the same: erratic data throughput that comes nowhere close to the performance levels expected from a channel containing a thermal noise component alone. In essence, the net spectral efficiency of the channel is degraded by the presence of interference.
Protect spectral efficiency
Although radio access technologies have made remarkable advancements in the last decade, the predominant methods for dealing with interference remain mired in the 1950s: when an impairment is noted (often the first alert is a customer complaint), a crew is dispatched to identify the interference source by triangulation, and, if possible, make arrangements for it to be switched off. While it is obviously desirable for interference sources to be eliminated when possible, this process is labor-intensive, slow, and leaves customers exposed to the effects interference while the response team scrambles to identify the issue. For difficult to resolve interference problems, this process can last for months – or indefinitely. Worse yet, less severe interference problems, although common, are often undiagnosed, resulting in a degradation of the aggregate spectral efficiency of the network. Remarkably, interference causing an unloaded noise rise of as little as 3 dB can result in reductions of area spectral efficiency approaching 25%. Fortunately, advances in adaptive RF digital signal processing (DSP) provide an avenue for implementing a more interference tolerant radio. By monitoring the RF signal for spectral distortions inconsistent with the expected signal, and adapting the channel in real time to compensate for the presence of any detected interference, the effects of impairments can be minimized or eliminated. Figure 1 illustrates the typical integration point for an external RF DSP. As is apparent from the diagram, the RF DSP can be thought of as an augmentation to the radio Rx path itself, that gives the radio the ability to adapt to the instantaneous RF environment.
RF DSPs: Under the hood
Interference can originate at arbitrary sources, so it can have arbitrary spectral signatures. However, since the spectral signature of the underlying air interface is known, departures from the expected power spectral density can be used to identify interference candidates1. Since RF power from multiple sources is present at the receiver, but only the characteristics of the desired signal are known, the interference components must be deduced by statistical methods. Hence, an adaptive system is comprised of two distinct components: a detection subsystem, capable of executing the required statistical analysis in real time, and a signal processing subsystem capable of changing the system’s frequency response in based on the decisions made in the detection process.
A simplified block diagram of a dual-channel RF DSP is shown in figure 2. An RF input is sampled by an ADC with dynamic range adequate to handle small signals (in the interference free scenario), as well as large interference powers. Detection algorithms then continuously monitor the characteristics of the input RF signal for the presence of interference. If any is detected, the system selfadapts its frequency response to provide the optimal signal-tonoise ratio at the output port, given the instantaneous interference- plus-noise signal present at the input. This optimized digital version of the input signal is then converted back to analog RF, and passed to the transceiver. This process, which may include filtering both within and outside the bandwidth allocated to the receiver’s air interface, is essentially conditioning the spectrum prior to demodulation at the radio.
RF DSPs in action
An example application for adaptive RF DSP is shown in figure 3. Here, three simultaneous, singletimeslot GSM bursts are injected co-channel to a victim UMTS uplink signal. This interference scenario often occurs at international borders, due to crossborder mobile station (MS) transmissions. It is also an interference scenario that must be managed by an operator when deploying UMTS in spectrum previously allocated to GSM.
GSM-as-interference is among the most difficult sources for adaptive RF DSPs to track, since due to the hybrid TDMA/FDMA structure of the air interface the signal is highly dynamic in both the frequency- and timedomains. The GSM burst must be acquired and attenuated in a timeframe short relative to its 577 μs duration, adequate attenuation must be provided to reduce the power over its 200 kHz modulation bandwidth, and multiple sources (at multiple frequencies) must typically be tracked simultaneously. In the example shown, three independent bursts are tracked and attenuated by approximately 20 dB each. This result is achieved by the RF DSP alone, and requires no synchronization with the interfering GSM network. In this example, the spectral efficiency benefits provided by the technology are most easily demonstrated by evaluating the link budget from the interfering GSM MSs to the victim Node B. As illustrated in figure 4, in a mixed suburban environment, UMTS and GSM networks operating in the same band must be kept 23 km apart to ensure that any GSM transmissions received at the Node B are below the thermal noise floor. The additional 20 dB of margin provided by the RF DSP allows the networks to be brought within 6 km of each other under the same constraints. The ability to deploy UMTS in such close proximity to GSM, in a geographic region in which the spectrum would otherwise have been unused, represents an enormous improvement in spectral efficiency – greater than 50% in this case.
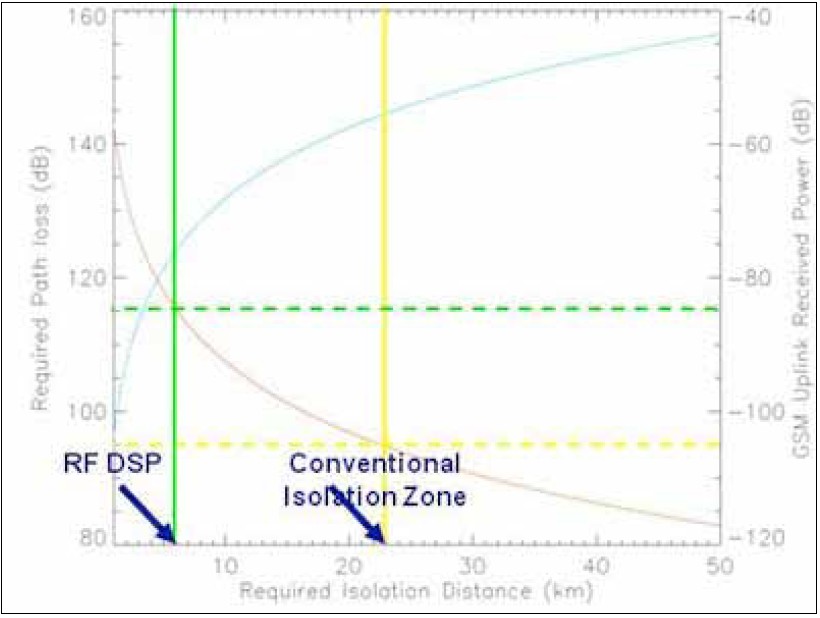
Figure 4: Spectral efficiency benefits provided by RF DSPs applied to the GSM-on-UMTS co-channel interference scenario. The required isolation distance between GSM and UMTS is reduced by 75%.
RF DSPs can also be used to improve adjacent channel selectivity (ACS). In figure 5, highly selective band-reject filters have been configured to provide additional isolation on the GSM channels operating at the smallest frequency offsets from the UMTS carrier frequencies. Since the filters are software configurable, here their frequencies and bandwidths have been set to match the channel frequencies and modulations envelopes, respectively, of the adjacent channel GSM signals. This ensures ACS is optimized for this specific application.
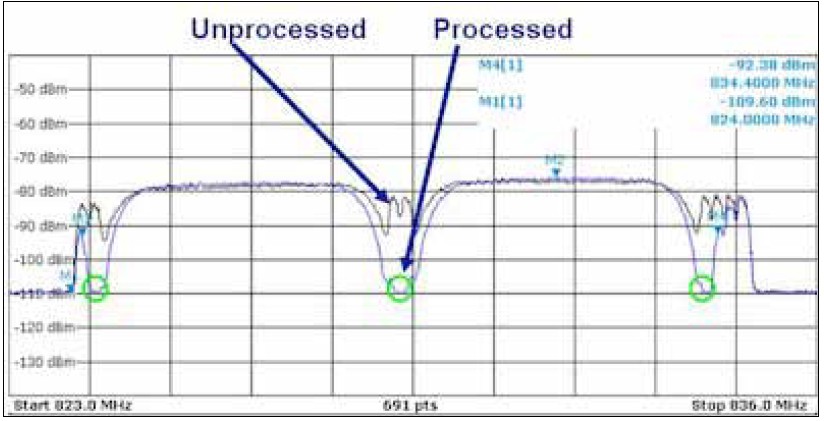
Figure 5: Attenuation of adjacent channel GSM interference with RF DSPs allows GSM to operate at narrower frequency offsets from UMTS.
This approach allows UMTS to be deployed in spectrum previously used for GSM, while minimizing the impact on the existing 2G network. The effectiveness of the filtering from the RF DSPs is such that the adjacent channel interference ratio (ACIR) is comparable to the case when the nearest adjacent channel is not used at all – allowing UMTS to be operated in the presence of GSM operating at small offsets with no penalty in UMTS capacity. Obviously, the use of radio channels that would otherwise be unavailable in this scenario is another direct demonstration of improvement of spectral efficiency by using smart RF DSPs to manage spectrum.
Spectrum awareness
Tuning information used to adapt the frequency response of the RF DSP in response to detected interference can be stored, which allows a repository of information regarding the interference environment to be built up in the course of operation. The information can then be downloaded and postprocessed, providing operational teams a powerful additional view into the RF environment in which their network is operating. This information can then be used to augment traditional interference tracking methods, when appropriate. In this case, while the response team acts on the interference information gathered by the device, the adaptive filter subsystem continues to protect the radio, providing uninterrupted high quality of service levels even in the face of severe interference.
An example of such data is shown in figure 6. Here, the time of the interference event is plotted against frequency, and interferer power is indicated by the false color scale on the right. This spectrogram view provides a powerful visual summary of the interference environment over a period of time in a single integrated view – and also, since the channel conditions are not static, clearly demonstrates the advantages of adapting the radio to the channel conditions. Deployed over a wide network area, the RF channel “situational awareness” offered by this technology provides a valuable information source for ensuring spectrum fidelity.
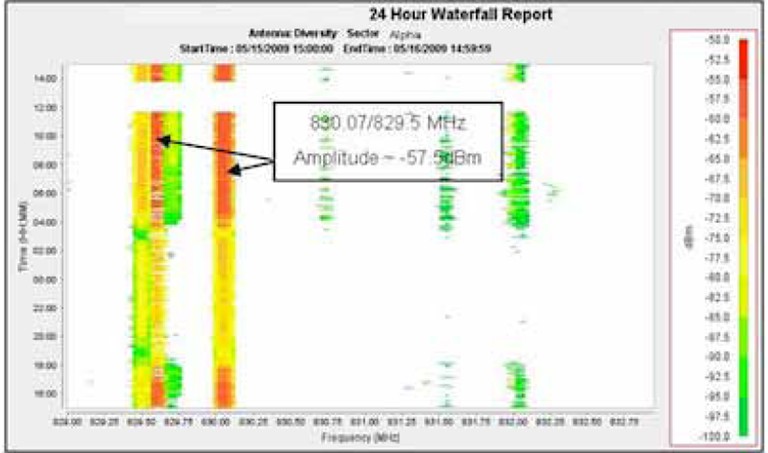
Figure 6: Spectrogram interference data gathered by an adaptive RF DSP system in a live site, illustrating the power and frequency variability of the interfering signals.
Spectrum conditioning
As the impending spectrum crunch approaches, the need to treat spectrum as a precious resource is becoming more acute. Additional spectrum allocations will be required to meet longterm demand, but maintaining high spectral efficiency across the spectrum allocated to terrestrial wireless services will be equally vital. Just as advances in air interface technologies have steadily improved the spectral efficiency of wireless networks, advances in RF DSP techniques have allowed adaptive RF to become a reality, and provide a method for maintaining high spectral efficiency, even in suboptimal and unpredictable real-world RF environments.